Dark energy and the fate of the universe
My research focuses on optimizing methods for detecting signatures of time-varying dark energy in observational data, as well as developing techniques for interpreting what those patterns tell us about the finer details of its evolution. By distinguishing between a cosmological constant and different forms of dark energy, we move closer to a true understanding of what the future has in store for our universe.
The standard model of cosmology assumes that the accelerated expansion of the universe is driven by a cosmological constant—a form of energy intrinsic to the vacuum, with a constant density throughout space and time. If this is the case, then the acceleration of the universe will continue indefinitely, eventually stretching space so much that all but the nearest galaxies will vanish from our sight.
On the other hand, if this acceleration is caused by a dynamic field of dark energy—one whose density evolves over time—then the expansion of space could stabilize, return to a decelerating trajectory, or even reverse into an era of cosmic contraction.
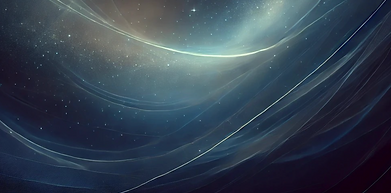
The universe before the Big Bang
Rather than being born in a singularity, it's quite possible that the universe has an infinite past. In many theories attempting to adapt the equations of General Relativity to higher temperatures, this infinite past is manifested by connecting the past 14 billion years of expansion with a preceding epoch of cosmic contraction. This possibility is extremely exciting, because it opens entirely new avenues for explaining conundrums at the heart of cosmology that have remained unsolved for the better part of a century.
Measurements of the chemical composition of the universe let us probe the state of the cosmos at very early times—all the way back to just one second after it started expanding. That's how we know that by the time the universe was one second old, it was already entirely thermalized, filled uniformly with plasma at a nearly constant temperature throughout. It was also spatially flat, isotropic, and void of magnetic monopoles. Decades have been spent trying to explain how these unusual properties could have been generated in less than a second of expansion through cosmic inflation, but the initial successes of these theories have been followed by credible concerns about fine-tuning, multiverses, and non-predictivity.
By leveraging the billions of years of contraction that may have preceded the current phase of expansion, cosmologists have proposed alternative explanations for the unusual state of the early universe that manage to avoid these concerns. My research has built on an alternative known as ekpyrotic contraction, in which the universe is permeated by a scalar field that gains a tremendous amount of energy during the contracting phase. The dynamics of this field cause the universe to flatten and thermalize, and the field later decays homogeneously into matter and radiation. While this type of theory comes with its own cosmological challenges, it is still in the early stages of development and there is much room left for exploration and innovation.
Using the equations of General Relativity, the observed expansion of the universe can be traced back 14 billion years into the past, through a regime where the universe was smaller, hotter, and denser (forming the basis of the Big Bang Theory), and culminating in a point of zero size and infinite temperature and density (the Big Bang Singularity). While the Big Bang Theory is backed by incontrovertible observational evidence, the Big Bang Singularity is not as much a realistic prediction of General Relativity as it is an indication that the equations become unreliable at high temperatures.
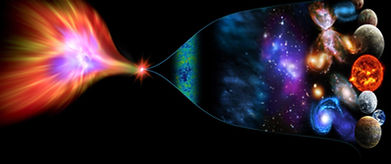
Cosmology informed by string theory
At the same time, explorations of string theory (and quantum gravity more generally) have led to a number of conjectures about the kinds of physics that we should not expect to observe in our universe. These so-called Swampland Conjectures suggest that a broad range of effective field theories that appear theoretically sound in the low-energy limit may actually be incompatible with an ultraviolet quantum-gravity completion.
From the perspective of a cosmologist, the Swampland Conjectures are useful for narrowing down the vast array of possible explanations for the homogeneity of the early universe and for its present-day acceleration. My research has shown, for example, that the Trans-Planckian Censorship Conjecture rules out the use of axion-like fields to drive cosmic inflation and additionally constrains axion-based models of dark energy.
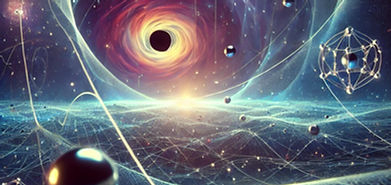
While string theory has not yet produced any concrete, testable predictions, it has certainly provided broader hints of the kinds of new physics we might expect to observe in our universe. One example is the presence of axion-like fields (pseudoscalar fields with periodic potentials), which arise generically as a consequence of compactification from the nine spatial dimensions of string theory to the three macroscopic dimensions in which we live. Depending on their mass and decay rate, axion-like fields can play many different roles in cosmology: They are a popular candidate for dark matter, and they could also (temporarily) drive a period of cosmic acceleration.